Learn about how the Magnitude Instruments inspIRe™ enables sensitive vibrational measurements of molecules bound to the surfaces of catalysts to study their excited state chemistry.
Enabling Studies of Excited State Surface Chemistry in Photocatalytic Systems
Table of Contents
ABSTRACT: Nanostructured semiconductors and quantum dots are attractive platforms for photocatalytic reactions that convert solar energy to fuels or that photodegrade environmental pollutants. Although molecular ligands on their surfaces mediate the electron transfer processes and chemical reactions that underpin photocatalysis, it has historically been challenging to study how changes in excited state surface chemistry affect photocatalytic reactions. Recent experimental advances from Magnitude Instruments now make it possible to directly examine excited state surface chemistry of nanostructured semiconductors, which opens new opportunities to guide the design of photocatalytic systems.
Excited state electronic processes underpin an array of photochemical reactions from photocatalytic conversion of sunlight to fuels to photodegradation of materials and environmental pollutants. Such processes convert energy from absorbed photons into electronic and nuclear motion to break and form chemical bonds. Nanocrystals and quantum dots (QDs) are attractive systems for light-driven photocatalytic reactions because of their strong light absorption properties and high surface areas, which enable electronic energy to be harnessed for charge transfer or chemical bond formation. Similarly, nanostructured or nanowire semiconductor systems decorated with catalytic reaction centers are being examined as modular platforms for photocatalysis. All of these photocatalytic systems involve chemical reactions at their surfaces that are driven by electronic energy from excited states of the nanoparticles. Therefore, experimental techniques capable of directly probing how the vibrational motions of molecules change in response to electronic excitation have long been needed to provide important insights regarding the photocatalytic pathways that underpin solar energy conversion to fuels and material degradation, among others.
Time-domain Raman and resonance Raman spectroscopy have been used to investigate vibrational motions of molecules coupled to the electronic transitions that are excited by photon absorption. 2-Dimensional electronic spectroscopy has also been used to reveal vibrational motions coupled to electronic processes by detection of coherent modulation of the electronic transitions. These methods struggle to provide information about the subsequent vibrational motions on longer time scales that lead to photochemical bond breaking and formation. Time-resolved infrared (TRIR) spectroscopy and femtosecond stimulated Raman spectroscopy (FSRS) are capable of directly probing the vibrational motions of molecules and materials following the initial electronic excitation processes. However, it has historically been challenging to measure vibrational motions of molecules and catalytic centers attached to nanomaterial systems in their excited states using these methods. This is because of the difficulty in the past of detecting small transient signals and the need to maintain moderate to low pump excitation conditions that avoid fast annihilation processes and thermal artifacts.
A recent paper published in J. Phys. Chem. Letters in 2020 demonstrates that it is now possible to directly examine changes in surface chemistry that occur in the excited states of nanoparticles by probing the time-dependent changes of the vibrational spectra of molecules bonded to their surfaces. The transient vibrational spectra appearing in Figure 1 were measured in PbS QD films using an inspIReTM mid-IR TA spectrometer, 20 ns after 532 nm excitation with 2 μJ/cm2 intensity.1 This excitation intensity led to ~0.01 excitons being absorbed per QD in the film, which is low enough to suppress annihilation processes and closely mimic operating conditions of photocatalytic systems. Importantly, the transient vibrational spectra reveal the vibrational features of oleate ligands attached to the PbS QD surfaces in their excitonic excited states (blue and black shaded regions in Figure 1A). The data indicate an overall shift from chelating ligand bonding geometries to more bridging and monodentate geometries (Figure 1B) in the excited state with corresponding weaker average Pb-O bonds at their surfaces. Because photocatalytic reactions occur at the surfaces of catalyst particles, the dynamic surface chemistry observed here suggests that surface bound molecules may experience increased mobility in the excited states of the nanoparticles. This in turn may enhance the ability of molecular species to undergo charge transfer processes and chemical bond rearrangements that underpin photocatalytic reactions.
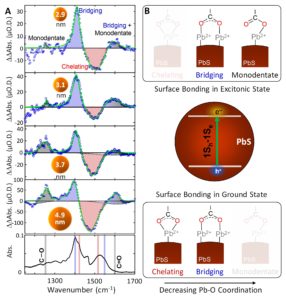
Figure 1. A Transient vibrational spectra of carboxylic groups bonded to PbS QD surfaces measured at 20 ns time delay following 532 nm excitation of films of PbS QDs having 2.9 nm, 3.1 nm, 3.7 nm, and 4.9 nm diameter and passivated with oleate ligands. The blue and gray shading highlights vibrational features of the oleate ligands attached to PbS QDs in their excitonic excited states. The red shading outlines the ground state bleach features. B. Cartoon depiction of the change in bonding from the ground to the excited excitonic states of the PbS QDs. The data reveal a decrease of the average Pb-O coordination in the excitonic excited states of the QDs, which could facilitate catalytic reactions at their surfaces. Adapted with permission from J. Phys. Chem. Lett. 2020, 11, 2291–2297. Copyright (2020) American Chemical Society.
REFERENCES
- Kennehan et al. J. Phys.Chem. Lett (2020) DOI: 10.1021/acs.jpclett.0c00539.
SHARE